Space Optical Communications: Why Are Space-to-ground Links Taking Time to Develop?
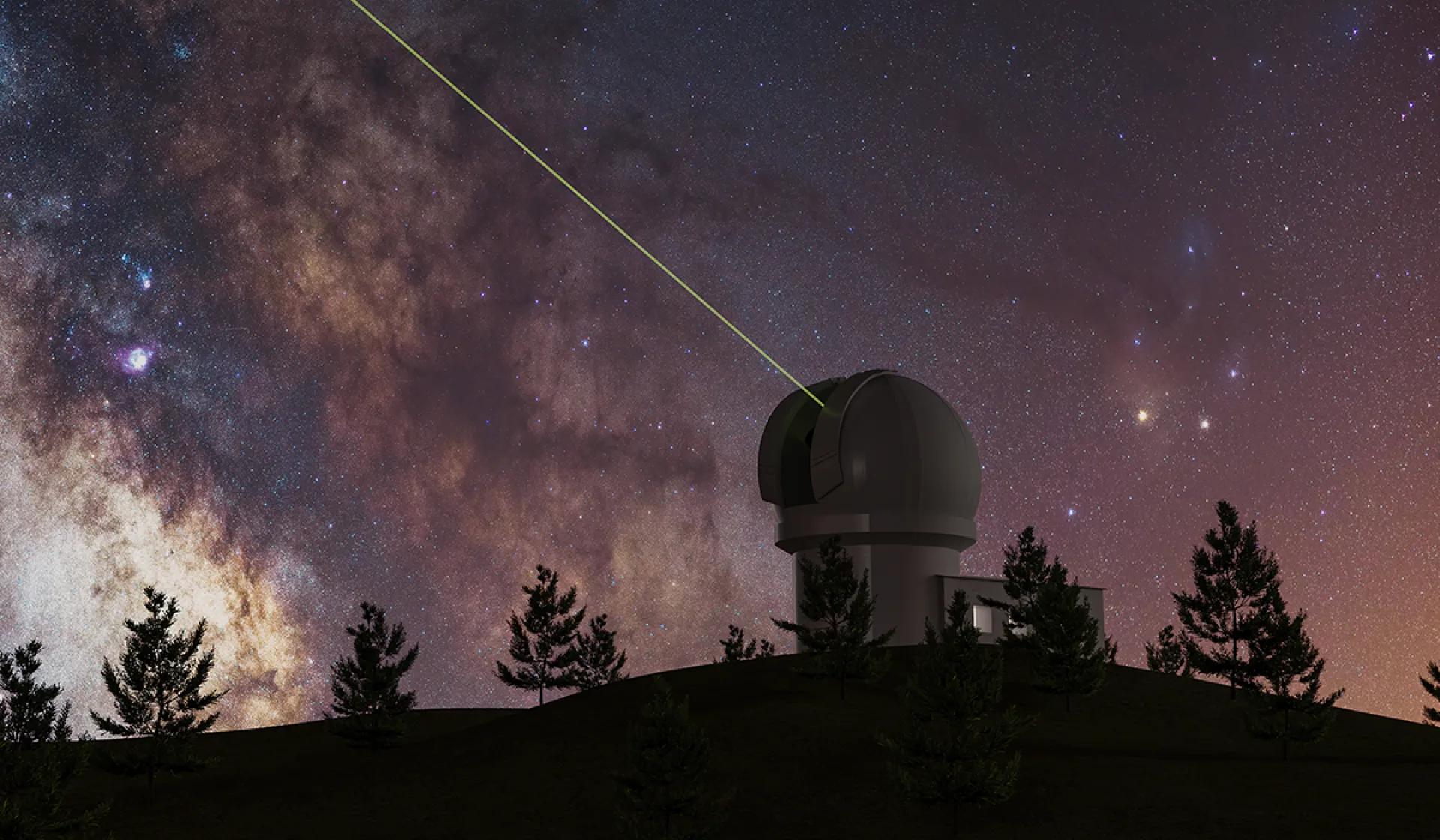
As seen in previous articles, a technological and market revolution in the space sector is reshaping the industry. Low Earth Orbit (LEO) satellite constellations are emerging as current radio frequency (RF) information transmission technologies are struggling to meet growing needs.
Attracted by its potential, actors push the development and adaptation of optical communications technology in the space infrastructure. Our previous article showed that many experiments have been carried out to prove the technical relevance of laser communication, but there is an imbalance in the way this technology is implemented for commercial missions.
Intersatellite links take off earlier than space-to-Earth
Within the scope of LEO satellite constellations, there are two main types of communications: the ones between space and Earth, and the ones between satellites, known as “inter-satellite”. In the context of commercial missions, this second type of link is developing faster than space-Earth communications, where RF is still preferred.
In fact, every new satellite in the Starlink constellation (SpaceX) put into orbit in 2022 or later will be equipped with a laser communication terminal. SpaceX, as the pioneer and symbol of the New Space movement, is closely followed by competitors that are planning a constellation of LEO satellites: OneWeb plans to integrate laser terminals, as well as Telesat. However, the objective of these terminals is indeed to establish inter-satellite links and not space-Earth links.
Structural reasons as the first part of the answer
In a legacy space communication infrastructure, for example composed of a geostationary satellite (GEO), the latter acts as a relay between two points of the main ground network. The information is sent from a first ground station to the satellite, which redirects it to a second receiver ground station on earth.
In this case, it is necessary that the satellite “sees” the two points on the ground simultaneously, in other words, the beams linking the satellite to the earth can effectively reach the ground stations. In the case of GEO satellites, this is generally not a problem, as one of them is generally able to effectively cover an area on the ground equivalent to 34% of the Earth’s surface. In the case of a transmission between two opposite points on Earth, the information can be transmitted by 2-3 satellites going back and forth between space and ground, keeping the main network on Earth.
However, things get complicated when we talk about LEO constellations. The latter orbiting at a much lower altitude (about 500/1000 km against 36000 km in GEO) the area potentially covered by one of them is much smaller. Moreover, from the ground they move in the sky at a very high angular velocity, making the small area on earth from which they are visible move very quickly.
To give an order of magnitude, a LEO satellite is visible from a fixed point on earth for 10-15 minutes (depending on altitude) and produces an instantaneous coverage area of about 2800 km radius. The last consequence of their orbit altitude is that they fly most of the time over oceans, and therefore cannot communicate with a terrestrial network.
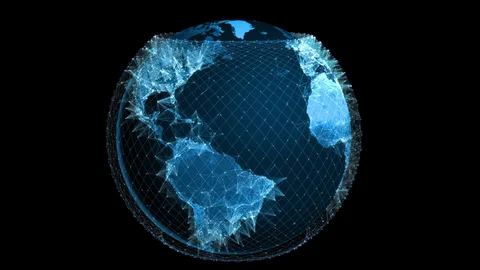
Thus, the structure of the network evolves at the same time these constellations appear: rather than having information pass back and forth between the satellites and the earth, the large number of terminals in orbit are used as nodes of a large network in the sky. The main network, the backbone, has thus been moved from the earth to space.
Optical communications become therefore particularly interesting to answer the need for high throughput capacity on this backbone when, for a simple individual user, RF is sufficient in terms of throughput and is currently easier to set up. In the case of Starlink, again, the user terminals are RF receivers. Satellite to ground optical links can become important in the case where the ground station is a relay to the end-users (one more intermediary than in the case of Starlink), which requires a higher throughput capacity.
Technical limitations have restrained space to ground optical links
In addition, some technical barriers to the implementation of optical links exist, contributing to the fact that RF is still largely dominant in the space-to-earth communications market today. Radio waves and light are both electromagnetic waves, having very distant frequencies. However, when light is emitted by a laser, it is concentrated in a beam directed in one direction and not very divergent, unlike radio transmitters which diffuse the wave in a much more open cone.
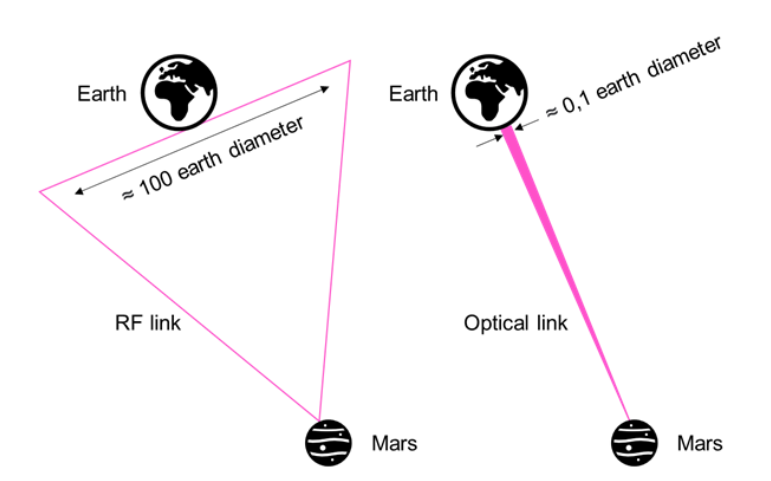
Thus, a first challenge lies in the precision of position pointing, the satellite must aim at the ground station on earth in a much finer way when optics is used compared to RF. In addition, the transmitting telescope onboard the satellite and the receiving telescope in the ground station must point at each other to align the beam in their respective axis.
As explained previously, LEO satellites move at high speed in the sky when seen from the Earth. This poses a second challenge with the pointing: the accuracy of the alignment between the transmitting and receiving telescopes must be maintained despite the high angular velocity observed between them. From the ground, this speed varies as the telescope moves in the sky: greater at the zenith, smaller at the horizon.
Finally, unlike inter-satellite communications, satellite to ground links go through the atmosphere. The differences in density, temperature, and humidity generate turbulence that degrades the quality of the signal (see Cailabs Academy). Thus, in the case of a LEO satellite, the strength of the technical difficulties alternates during the course of the latter in the sky: at the zenith the speed is higher, and at the horizon the distance of the atmosphere crossed is greater, increasing the impact of atmospheric turbulence.
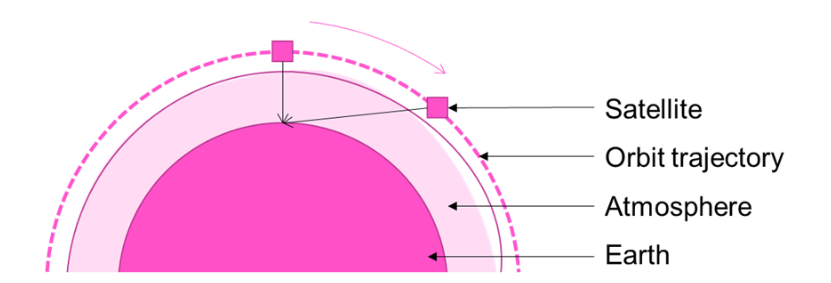
through atmosphere layerswith various thicknesses
There are solutions to overcome these technical limitations
However, the potential of optics in terms of throughput and communication sovereignty encourages the development of solutions to overcome technical difficulties. The low divergence of the laser beams poses a technical difficulty but also constitutes an advantage over radiofrequency because of the quasi uninterceptability of the link.
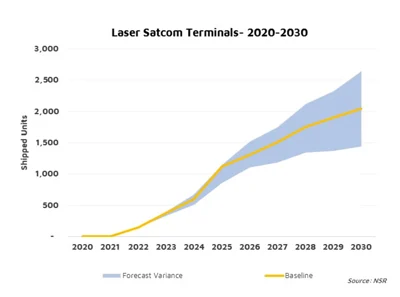
The difficulty on the pointing can be removed thanks to the use of telescope mounts inherited from astronomy, basically designed to compensate for the earth’s rotation for celestial objects observation. Knowing the position of the of both satellite and ground station allows this mount to perform a pre-positioning, which is refined using pointing auxiliary beams called “beacons”.
Astronomy has also bequeathed a technology for atmospheric turbulence compensation: adaptive optics. Originally implemented in large astronomy telescopes on earth to compensate for the effect of turbulence on observed images, its use has been extended to optical communications.
This solution, although suitable for use in some astronomy observatories with well-controlled environmental conditions, is not very suitable for optical communication ground stations. Indeed, the adaptive optics systems are very bulky, sensitive to vibrations, and have a relatively high price.
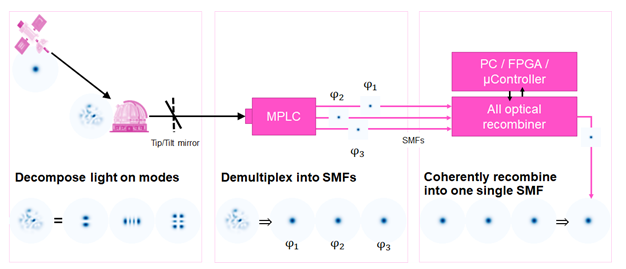
The use of another technology, the MPLC (Multi-Plane Light Conversion) can be much more attractive and suitable. Unlike adaptive optics, this technology has no moving parts and is much more compact, making it a robust and industrial solution. Thanks to this technology, Cailabs aims to push the development of the ground segment of optical communications, by allowing the compensation of atmospheric turbulence with a simple and effective component: TILBA®-ATMO. The integration of this component contributes to make unique the optical ground stations TILBA®-OGS designed by Cailabs.
Conclusion
As seen in the four articles of this blog articles serie, the space market is evolving at a high pace because of the concordance of disruptive innovations, the entry of private actors, and new ways to create value. This is of course impacting the whole network architecture and the communications channels have to adapt. In this context, laser communications seem to be relevant and will be necessary to satisfy the needs of throughput.
Many experimentations have been carried out, and commercial missions are now boarding laser terminals. Nonetheless, the optical communications have not yet rolled out their full potential, and the ground segment is still to be developed. Since there is no doubt this technology will be part of the space communication of the future, opportunities are to be seized on the ground segment.
Cailabs is offering turbulence mitigation solutions with its TILBA®-ATMO component, but also intents on taking up a leading position in the ground segment development with the design and manufacturing of industrial optical ground stations.
A first optical ground station have been built in 2022 as part of the Keraunos project, and SSC trusted in Cailabs for the realization of its first OGS. These projects pave the way to many more initiatives and demosntrate the growing interest around optical communications.
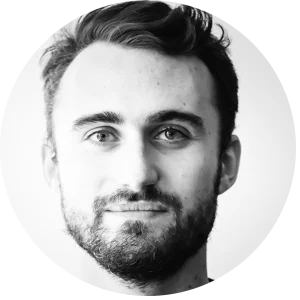
By Julien Bayol
Julien Bayol holds a Master’s degree in mechanics from INSA and has completed a Master’s degree in Management at Toulouse Business School. Very interested in advanced manufacturing techniques, through the use of innovative optical solutions, Julien is assistant product manager at Cailabs. He contributes to the development and commercialization of CANUNDA and TILBA® products.
Our Other Articles:
-
Exceeding Throughput Limits with Laser Communications
-
The challenges of e-mobility: Welding busbars
-
Flying further with beam shaping
-
From the Volkswagen Golf to the Tesla Model 3: How Lasers Are Shaping the Automotive Industry?
-
Space Optical Communications: Why Are Space-to-ground Links Taking Time to Develop?